5 Types of Hydrogen Storage
Hydrogen Storage | Compressed Gas Storage | Metal Hydride Storage | Chemical Hydride Storage | Liquid Hydrogen Storage | Cryo-Compressed Storage
HYDROGEN STORAGE
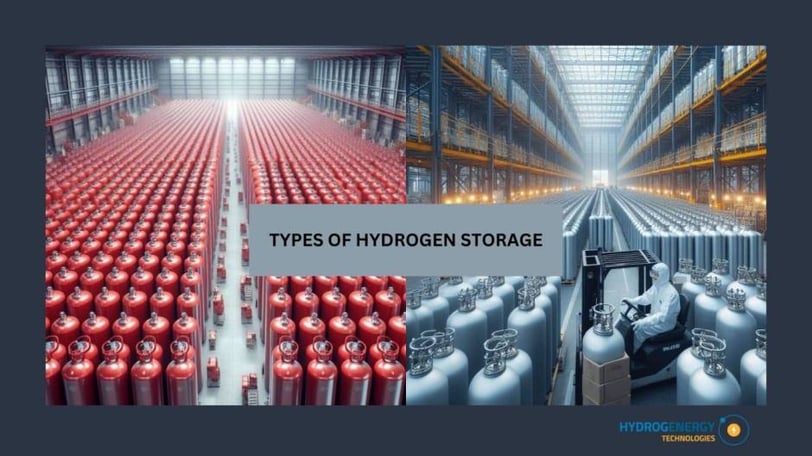
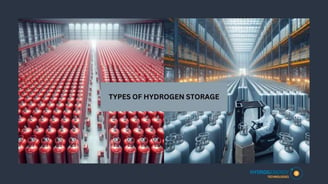
Hydrogen, recognized for its potential as a clean energy carrier, necessitates effective storage solutions to facilitate its widespread adoption in various sectors, including transportation, industry, and power generation. This blog delves into the primary 5 types of hydrogen storage methods—compressed gas, liquid hydrogen, metal hydrides, chemical hydrides, and cryo-compressed hydrogen—examining their advantages and disadvantages to provide a comprehensive understanding for students, researchers, and professionals in the hydrogen and net zero industry.
1. Compressed Gas Storage
Overview: Compressed gas storage involves storing hydrogen gas in high-pressure cylinders. The typical storage pressures range from 350 to 700 bar (5,000 to 10,000 psi), with advancements in materials technology allowing for higher pressures.
Advantages:
Simplicity and Maturity: Compressed gas storage technology is well-understood and commercially available, making it a straightforward option for hydrogen storage.
Scalability: This method is scalable from small portable cylinders to large-scale storage systems, enabling diverse applications.
Efficiency: Compression systems can be highly efficient, with well-established processes for compressing and decompressing hydrogen.
Disadvantages:
Energy Intensive: Compressing hydrogen to high pressures requires significant energy input, which can impact the overall efficiency and cost-effectiveness of the storage system.
Safety Concerns: High-pressure storage poses safety risks, including the potential for leaks and explosions, necessitating robust safety measures and monitoring systems.
Material Challenges: Storage vessels must be constructed from materials that can withstand high pressures and prevent hydrogen embrittlement, which can lead to material degradation over time.
2. Liquid Hydrogen Storage
Overview: Liquid hydrogen storage involves cooling hydrogen gas to cryogenic temperatures (approximately -253°C or -423°F) to convert it into a liquid state. This method significantly increases hydrogen's volumetric energy density.
Advantages:
High Energy Density: Liquid hydrogen has a higher energy density compared to compressed gas, enabling more hydrogen to be stored in a given volume.
Transportation Efficiency: The higher density of liquid hydrogen facilitates more efficient transportation, especially over long distances.
Established Technology: Liquid hydrogen technology is well-established, particularly in the aerospace industry, where it has been used as rocket fuel.
Disadvantages:
Cryogenic Temperatures: Maintaining hydrogen at cryogenic temperatures requires sophisticated insulation and refrigeration systems, leading to higher costs and energy consumption.
Boil-off Losses: Hydrogen can evaporate (boil-off) during storage and transportation, resulting in losses and necessitating continuous cooling.
Infrastructure Requirements: Liquid hydrogen storage and handling infrastructure are more complex and expensive compared to compressed gas systems.
3. Metal Hydride Storage
Overview: Metal hydrides involve storing hydrogen within a metal lattice, where hydrogen molecules dissociate into atoms and are absorbed by the metal. Common metal hydrides include magnesium hydride and sodium alanate.
Advantages:
High Density: Metal hydrides can store hydrogen at higher densities compared to compressed gas and even some liquid hydrogen systems.
Safety: Hydrogen stored in metal hydrides is chemically bound, reducing the risk of leaks and explosions.
Reversibility: Many metal hydride systems offer reversible hydrogen absorption and desorption, enabling repeated use.
Disadvantages:
Weight: Metal hydride storage systems tend to be heavier, which can be a disadvantage for mobile applications such as vehicles.
Thermal Management: Absorption and desorption of hydrogen in metal hydrides are exothermic and endothermic processes, respectively, requiring effective thermal management systems.
Material Cost: The metals used in hydrides can be expensive, impacting the overall cost of the storage system.
4. Chemical Hydride Storage
Overview: Chemical hydrides store hydrogen through chemical reactions, typically involving compounds such as sodium borohydride or ammonia borane. Hydrogen is released through hydrolysis or thermal decomposition.
Advantages:
High Storage Density: Chemical hydrides can offer high hydrogen storage densities, making them suitable for portable and stationary applications.
Stability: Chemical hydrides are generally stable at ambient conditions, reducing the need for high-pressure or cryogenic storage.
Ease of Handling: Many chemical hydrides are easy to handle and transport in solid or liquid form.
Disadvantages:
Hydrogen Release: Releasing hydrogen from chemical hydrides often requires additional processes, such as heating or reacting with water, which can be energy-intensive.
Regeneration: Regenerating spent chemical hydrides for reuse can be complex and costly, impacting the overall sustainability of the storage system.
Waste Management: Byproducts generated during hydrogen release need to be managed and recycled, adding to the system's complexity.
5. Cryo-compressed Hydrogen Storage
Overview: Cryo-compressed hydrogen storage combines the principles of compressed gas and cryogenic liquid storage. Hydrogen is stored at cryogenic temperatures and under pressure, maximizing its volumetric energy density.
Advantages:
Enhanced Density: This method achieves higher hydrogen density compared to standalone compressed or liquid hydrogen storage.
Reduced Boil-off: By combining pressure and cryogenic temperatures, cryo-compressed storage minimizes boil-off losses.
Efficiency: Cryo-compressed systems can be more energy-efficient, leveraging the benefits of both high-pressure and low-temperature storage.
Disadvantages:
Complexity: Cryo-compressed storage systems are complex, requiring advanced materials and insulation technologies to maintain both pressure and cryogenic temperatures.
Cost: The complexity of cryo-compressed systems results in higher costs for both infrastructure and operation.
Thermal Management: Effective thermal management is critical to prevent temperature fluctuations and maintain system integrity.
Conclusion:
The choice of hydrogen storage method depends on specific application requirements, including storage capacity, safety, cost, and infrastructure compatibility. Each storage method—compressed gas, liquid hydrogen, metal hydrides, chemical hydrides, and cryo-compressed hydrogen—offers distinct advantages and disadvantages. Understanding these factors is crucial for optimizing hydrogen storage solutions to support the transition to a sustainable, hydrogen-based energy economy.
Keywords: hydrogen storage methods, energy transition, sustainable energy, storage capacity, application requirements
By analyzing the pros and cons of each hydrogen storage technology, stakeholders can make informed decisions to advance the adoption of hydrogen as a clean energy carrier.
View our product page to purchase Compressed and Metal Hydride Storage