What is MEA in PEM fuel cell?
What is Membrane Electrode Assembly in PEM Fuel Cell? How is works? What are it's sub-components? What its is made up of and future prospects?
FUEL CELL
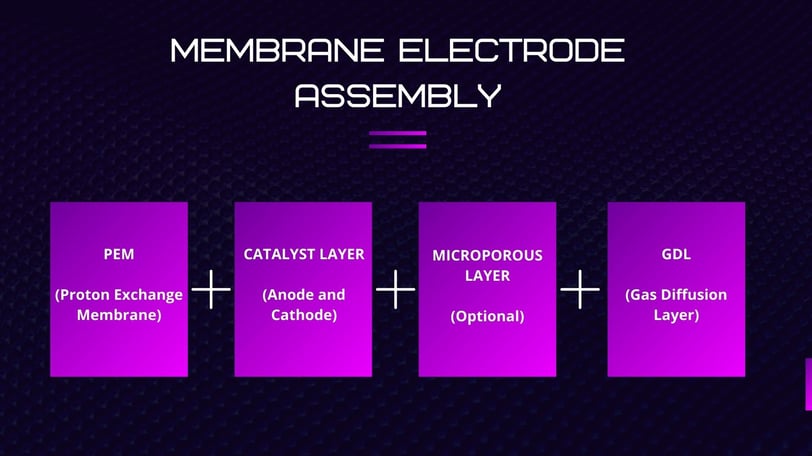
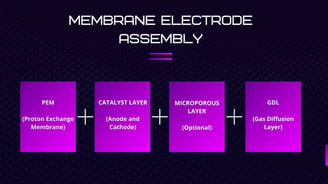
Understanding MEA (Membrane Electrode Assembly) in PEM Fuel Cells
Fuel cell technology represents a significant advancement in the quest for cleaner and more efficient energy sources. Among the various types of fuel cells, the Proton Exchange Membrane (PEM) fuel cell stands out due to its potential for automotive applications and other mobile uses. Central to the operation of a PEM fuel cell is the Membrane Electrode Assembly (MEA). This article will delve into the details of how the MEA works, the materials used in its construction, and its role in the overall function of the fuel cell.
Components of the MEA
The MEA is a multi-layered structure composed of the following parts:
Proton Exchange Membrane (PEM)
Catalyst Layers (Anode and Cathode)
Microporous Layers (optional)
Gas Diffusion Layers (GDL)
1.Proton Exchange Membrane (PEM)
The PEM is a polymer electrolyte membrane that allows protons (hydrogen ions) to pass through while being impermeable to gases like hydrogen and oxygen. The most commonly used material for PEMs is Nafion, a sulfonated tetrafluoroethylene-based fluoropolymer-copolymer.
Key Properties and Materials:
Proton Conductivity: High proton conductivity is essential. Nafion achieves this due to its sulfonic acid groups that facilitate proton transfer.
Chemical Stability: It must withstand the harsh oxidative environment within the fuel cell. Nafion's fluoropolymer backbone provides excellent chemical stability.
Mechanical Strength: The membrane needs to be durable enough to handle pressure and stress. Nafion is mechanically robust, though additional reinforcement (like expanded polytetrafluoroethylene, or ePTFE) can enhance its strength.
2.Catalyst Layers
On either side of the PEM are the catalyst layers, which consist of finely dispersed platinum or platinum-based alloys on carbon support. These layers facilitate the electrochemical reactions necessary for the fuel cell to generate electricity.
Materials:
Platinum (Pt): Used because of its excellent catalytic properties. Sometimes alloyed with other metals (e.g., ruthenium, palladium) to enhance performance and reduce poisoning by impurities.
Carbon Support: Typically Vulcan XC-72, a type of carbon black, which provides a high surface area for the platinum particles to disperse, enhancing the reaction efficiency.
Anode Catalyst Layer: Where hydrogen gas is oxidized.
Cathode Catalyst Layer: Where oxygen gas is reduced.
3.Gas Diffusion Layers (GDL)
The GDLs are situated adjacent to the catalyst layers and serve multiple purposes:
Facilitate Gas Transport: They ensure even distribution of hydrogen and oxygen to the respective catalyst layers.
Conduct Electrons: Made from carbon fiber, GDLs conduct electrons away from the reaction sites to the external circuit.
Manage Water: They help manage water produced at the cathode to prevent flooding and ensure the membrane remains hydrated.
Materials:
Carbon Fiber Paper or Cloth: Used for its high electrical conductivity and porosity.
Teflon (PTFE) Coating: Often applied to the GDL to make it hydrophobic, aiding in water management.
4.Microporous Layers (Optional)
Some MEAs include a microporous layer between the catalyst layer and the GDL. This layer can improve water management by preventing excessive water buildup and ensuring uniform distribution of reactants.
Materials:
Carbon Black and PTFE Mix: Applied as a thin layer to provide additional porosity and hydrophobicity.
Working Principles of the MEA
The MEA is where the core reactions of the PEM fuel cell occur. The process can be divided into several steps:
Hydrogen Oxidation at the Anode:
Hydrogen molecules (H₂) enter the anode side of the fuel cell.
The catalyst (typically platinum) facilitates the separation of hydrogen molecules into protons (H⁺) and electrons (e⁻).
The half-reaction at the anode is:
H2→2H++2e−H2→2H++2e−
Proton Transport Through the PEM:
The PEM allows protons (H⁺) to migrate from the anode to the cathode.
The membrane prevents the passage of electrons, forcing them to travel through an external circuit, creating an electric current.
Oxygen Reduction at the Cathode:
Oxygen molecules (O₂) enter the cathode side of the fuel cell.
The catalyst at the cathode facilitates the reaction of oxygen with the incoming protons and electrons to form water (H₂O).
The half-reaction at the cathode is:
12O2+2H++2e−→H2O21O2+2H++2e−→H2O
Overall Reaction:
The overall reaction in a PEM fuel cell can be summarized as:
H2+12O2→H2O+Electricity+HeatH2+21O2→H2O+Electricity+Heat
Detailed Breakdown of the Electrochemical Reactions
Understanding the electrochemical reactions in detail helps in grasping how the MEA operates within the PEM fuel cell.
Anode Reaction Mechanism
Adsorption: Hydrogen molecules adsorb onto the platinum catalyst surface.
Dissociation: The H₂ molecules dissociate into individual hydrogen atoms, which then ionize to form protons and release electrons.
Desorption: Protons are desorbed from the catalyst surface and migrate through the PEM.
Cathode Reaction Mechanism
Adsorption: Oxygen molecules adsorb onto the platinum catalyst surface at the cathode.
Dissociation: The O₂ molecules dissociate into individual oxygen atoms.
Reduction: The oxygen atoms combine with protons and electrons to form water molecules.
Challenges in MEA Development
Despite their potential, PEM fuel cells face several challenges related to the MEA:
Cost of Catalysts: Platinum is expensive and its high cost remains a significant barrier.
Durability: The MEA must be durable to withstand the operational stresses over time, including the harsh chemical environment and mechanical stress.
Water Management: Effective water management is crucial to avoid both flooding and dehydration of the membrane.
Thermal Management: The heat generated during the reaction needs to be effectively managed to maintain optimal operating temperatures.
Advancements in MEA Technology
Ongoing research and development are focused on addressing these challenges:
Catalyst Innovations: Research is aimed at reducing platinum loading, finding alternative materials, and improving catalyst durability.
Non-Platinum Catalysts: Development of catalysts based on materials such as transition metal carbides, nitrides, and oxides.
Platinum-Alloy Catalysts: Alloys with reduced platinum content but enhanced catalytic activity.
Membrane Improvements: New materials and composites are being developed to enhance proton conductivity, chemical stability, and mechanical strength.
Aquivion and Other Ionomers: Alternatives to Nafion with improved conductivity and durability.
Composite Membranes: Combining polymers with inorganic materials for enhanced performance.
Enhanced GDLs: Innovations in the design and materials of GDLs are improving gas diffusion and water management.
Advanced Carbon Materials: Use of carbon nanotubes and graphene to improve conductivity and durability.
Hydrophobic Treatments: Enhanced PTFE or other hydrophobic coatings for better water management.
Integrated Water and Thermal Management: Advanced designs are incorporating better water and thermal management systems to enhance performance and longevity.
Microfluidic Channels: Precision-engineered pathways for optimized water and thermal regulation.
Heat Exchangers: Improved designs for efficient heat dissipation.
Future Outlook
The future of PEM fuel cells and their MEAs is promising, especially with the push for sustainable energy solutions. Key areas of focus include:
Cost Reduction: Through material innovations and economies of scale, the cost of PEM fuel cells is expected to decrease.
Performance Enhancement: Continuous improvements in MEA design and materials will lead to higher efficiency and durability.
Mass Adoption: As technology matures, PEM fuel cells could see widespread adoption in various sectors, particularly in transportation and portable power applications.
Interested in buying fuel cells : Explore our products